Chapter 5: Water Balance Components
Describes the field techniques used to measure the principal water balance mass components and determine their solute and isotopic values. The decision to measure evaporation independently of the thermal balance required that a floating pan system be designed and engineered. The literature on floating pans is generally concerned with very large raft mounted installations. The design adopted for Perry Lakes included a bird guard with minimal wind and solar shading, stability without the use of rafts, provisions to increase floatation when heavy rain was forecast, a simple mechanism to level and stabilise the pan during readings and a wave damping mechanism.
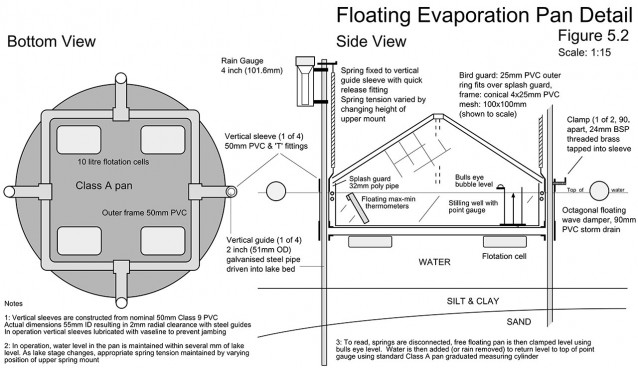
Details of the floating pan designed and built specifically for Perry Lakes. One of the greatest disadvantages of floating pans is the fact that during heavy rain, the pan fills and rides lower in the water. High winds invariably associated with major frontal systems increase the likelihood of wave slop both into and out of the pan. In the Perry Lakes design these problems were circumvented in two ways. During winter when a frontal passage was forecast, spring tension was increased. This has the immediate effect of giving the pan greater freeboard, and preventing slop in. The pan was also equipped with an anti-slop ring or 'splash guard' around its rim and was surrounded by a floating wave damper. If significant rain was also forecast (>20mm), the water level in the pan was also lowered by 20-30mm prior to the frontal passage, preventing slop out. The spring assisted guides allowed the pan to move vertically through at least 0.5m in any 24 hour period, this being the typical lake stage change from storm water or summer top up. Over 13 months of continuous daily operation, only two days data were lost, once from flooding during an extreme rain event and once from flooding when the pan was inadvertently left in the 'locked' position after being read. Daily evaporation was read to 0.1mm using a standard Class A pan graduated measuring cylinder and adjusted for rainfall collected at the pan site.
Storm water enters Perry Lakes via 7 major drains and represents a significant component of the water balance. At first glance the problem appears simple. If the pipes are long, straight, debris free and of constant diameter, gradient and construction, then empirical techniques such as those derived by Chezy and Manning can provide useable estimates of open channel flow in pipes using simple empirical equations. This was the approach adopted at West Lake where 2 large drains were equipped with acoustic loggers which bounce an ultrasonic acoustic signal off the water surface and use the time delay of the returning signal to calculate distance to the water surface.
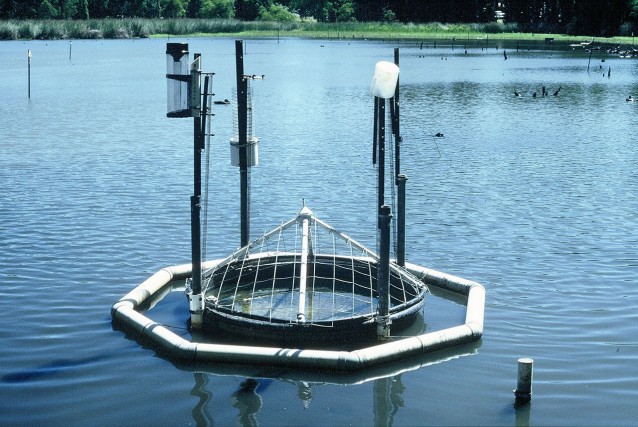
Floating Class A evaporation pan in operation. This simple yet rigorous and independent measure of lake evaporation allowed us to resolve the sediment heat flux, a component usually ignored and demonstrate that in shallow wetlands and lakes, sediment heat flux is a significant component of the thermal balance.
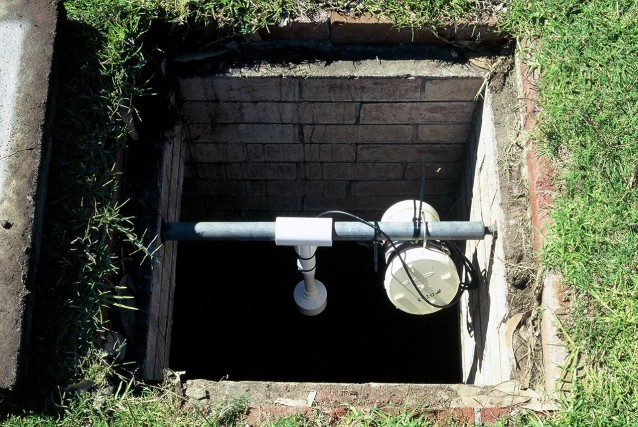
Acoustic logger mounted in saddle trap over 920mm diameter storm drain, West Lake. The instrument could operate continuously for 2 months with readings every minute. Water depth could be resolved to +/- 1mm.
Drains entering East Lake varied from 225mm to 680mm diameter and presented significant difficulties. None provided access to undisturbed flow (i.e. West Lake style saddle traps). Instead they were built with square sediment traps. Generally several pipes entered the last trap, with a single pipe of larger diameter entering the lake. Observing these traps during storm events it was evident that there was a relationship between water level in the traps and water level in the exit pipe. The solution was to equip all sediment traps with capacitive water level loggers and then design an instrument to calibrate the simultaneous relationship between water depth in the trap and depth in the exit pipe.
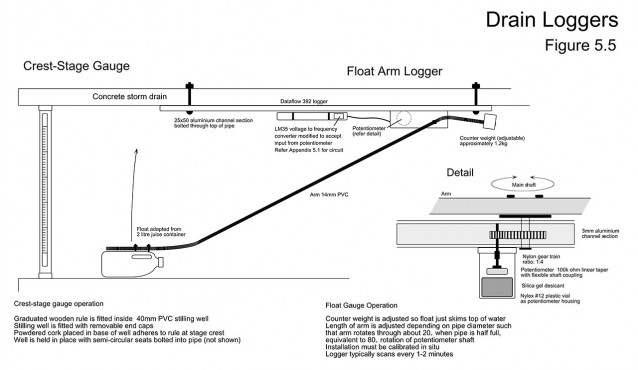
It was clear by the end of winter 1996 that the electronic data being collected in the sediment traps could not be adequately calibrated using either direct measure or crest-stage gauges. The final solution was to design and build a sensor which would continuously monitor water height in a pipe and log it electronically over short (minute or two minute) intervals. Data from one or two major storm events would be sufficient to calibrate the sediment trap loggers. The final design consisted of a counterweighted float and arm driving a potentiometer via a 1:4 gear train. The arm length was adjusted (depending on pipe diameter) such that flow at 0-90% of pipe diameter moved the arm through about 20° of rotation (thus maintaining the float horizontal to the water surface). Movement through this small rotational angle was multiplied via the gear train driving a potentiometer to provide increased resolution of small changes in depth. The counterweight was adjusted such that the float just skimmed the top of the water. A voltage to frequency converter and standard data logger were modified to accept input from the potentiometer.
Chapter 5 is a practical demonstration of how research often requires innovation and a bit of ‘hands on’ engineering skill. Both the floating pan and float arm drain logger were built from simple materials and cost very little. There was simply no commercially available gear that could have done the job.