To determine the isotopic water balance of a lake the critical parameters are δE, the isotopic composition of water vapour evaporating from the lake surface and δA, the isotopic composition of water vapour in the local air mass. In many such studies δE is simply estimated using empirical formulae. These in turn require knowing (among other things) δA and δL, the isotopic composition of the lake water. Again δA is often simply estimated from the literature. At Perry Lakes the isotopic value of each balance component was measured directly. This included rainfall (each rain event), lake water (every 4 days) and top up water (each time groundwater was pumped into the lake).
Due to the time and expense involved in measuring δA little detail is known regarding the isotopic composition of water vapour generally over Australia. In general δA varies diurnally (primarily in response to humidity) and seasonally in response to varying air mass provenance. At Perry Lakes δA was measured directly on a weekly basis by freezing out the water vapour in traps chilled to -60 degrees C. A total of 108 samples and δA determinations were completed over the study period.
Evaporation pans can be used to estimate δE. The literature describes two basic approaches. In the first pans are allowed to approach dryness. Water in the pan then approaches a limiting steady state composition designated δS. In the second approach pans are evaporated at constant volume i.e. as water is evaporated from the pan it is replaced with water of a known isotopic composition. The water in such pans will also reach a limiting steady state (designated δK). Both δS and δK vary with humidity and δA. When pans are evaporated over days or weeks, the steady state values reflect the prevailing average atmospheric conditions including humidity and δA. The resulting steady state values are said to be ‘flux weighted’ in that they reflect the sum effect of the varying exchange and evaporative processes occurring over time. If such pan experiments are conducted continuously over a year, the resulting steady state compositions of the pan water will constantly change, reflecting the seasonal variations in humidity, δA and other atmospheric conditions. If such experiments are conducted beside a lake and if the water in the pans is subjected to the same atmospheric conditions as the lake, then δS and δK measured in the evaporation pans can be used to estimate δE for the adjacent lake.
The Perry Lakes pan exchange parameters experiments comprised two small pans mounted within larger thermal regulation pans. Water from the adjacent lake was continuously pumped into these outer pans so that their temperature was always equal to that of the lake. The isotope experiment ran continuously from March 29, 1996 until February 14, 1998 and comprised 20 separate time course runs (20 for each pan, 40 in all) over 688 days or almost two years of flux weighted evaporation data. Mean run time was 34 days.
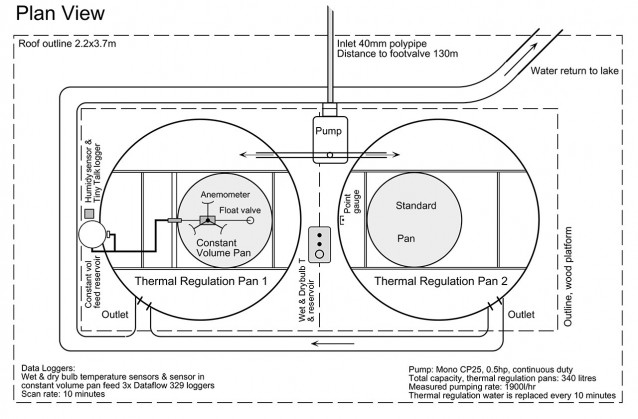
Fig. 12.4 Plan View
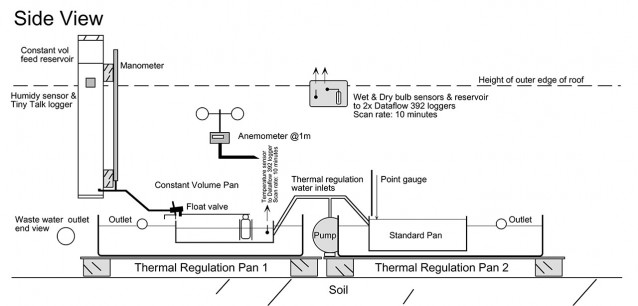
Fig. 12.4 Side View
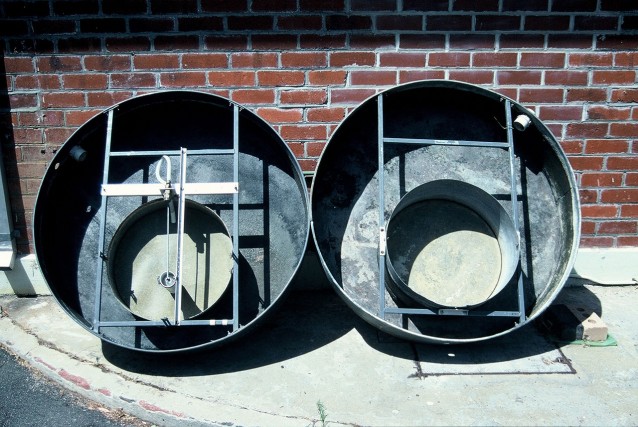
Basic pan experiment layout, photographed after pans were decommissioned, February 1998. The experiment pans are mounted eccentrically within the Class A thermal regulation pans. The eccentric mount pattern kept the pans close to the centre of the shelter, precluding rain contamination of the experiments. Lake water circulated through the outer pans at about 1900 litres/hr and kept the experiment pans within 1 degree C of lake surface temperature.
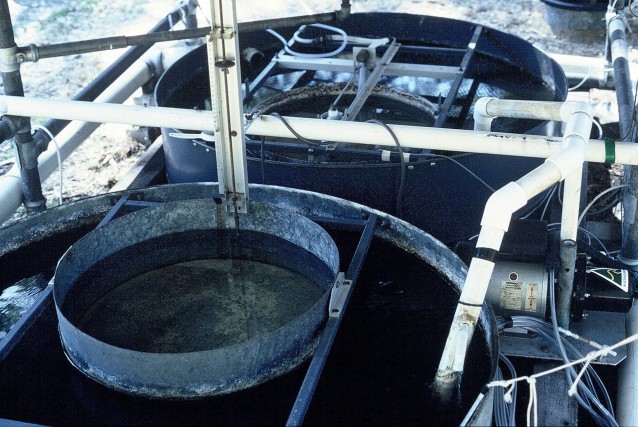
Pan evaporated to dryness. The daily evaporation from the pan was read using a ‘point gauge’ visible behind. It comprised a threaded brass rod machined to a fine point plus a brass disk mounted on the rod whose position could be sighted against a fixed scale allowing evaporation to be read directly in mm. In operation the rod was screwed down until the tip contacted the water surface, with evaporation then read directly off the scale.
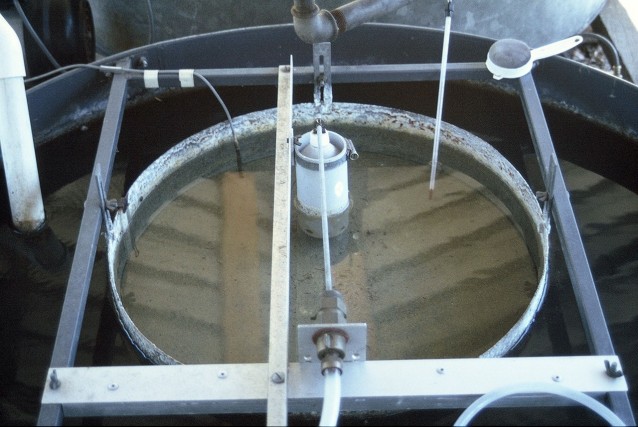
Pan evaporated at constant volume. Thermal regulation water enters outer pan via the white pipe on the left. As evaporation proceeds, the water level in the pan is maintained constant via the float (in stilling well) and valve (foreground). Thermistor (left rear) connects to data logger, checked daily against a glass laboratory thermometer, (right rear). The float and valve were fed by a small volume, manometer equipped reservoir built from PVC pipe. Daily evaporation could be read to 0.1mm accuracy.
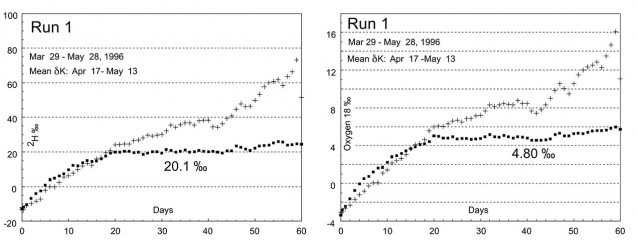
Typical deuterium time course for pan evaporated at constant volume (rectangles) and pan evaporated to dryness (+ symbols). This first run was allowed to run longer than necessary. At the height of summer steady isotopic state was frequently achieved in the constant volume pan in about 12 days. Constant volume steady state (δK) can be read directly, in this case 20.1‰. Oxygen 18 time course is also shown for the same period. Initially pans were sampled and analysed every day (Runs 1-11) while Runs 12-20 were analysed every second day. Water evaporated in the pans was groundwater of similar isotopic composition to water naturally discharged into East Lake under flow-through conditions.
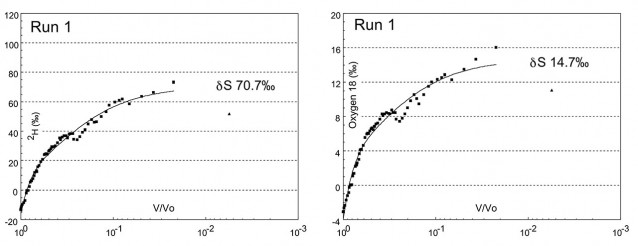
Determination of steady state (δS) for deuterium in pans evaporated to dryness. The x axis is the ratio of pans volume V at time t over initial volume V0 at time t0. Steady state δS is the value of the curve extrapolated to V/V0 = 0.0001. Second graph shows similar data for Oxygen 18. The final data point (plotted as a triangle in both graphs) is ignored. As dryness is approached salt in the pan water crystallises and absorbs atmospheric moisture corrupting the final value.
The Perry Lakes isotopic balances are unique in that they employ isotopic exchange parameters measured directly at the study site (such as δA) or determined experimentally specifically for the study site (δS, δK and δE).
Chapter 12 ends with details of experiments carried out to measure δE directly. On cold still mornings at Perry Lakes vapour is frequently visible rising from the warmer water into the cooler air (refer Home Page). This is evaporated lake water vapour, made temporarily visible through condensation. The vapour only becomes visible some distance above the lake surface. Immediately above the lake surface the air is warmed by the water and no vapour is visible. We postulated that if air from this layer could be sampled without allowing any fractionation, it would provide a means of directly measuring the isotopic composition of water vapour evaporating from the lake surface, δE. A floating sampler was devised (refer photo). The vapour was collected by freezing it out in traps chilled to -60 degrees C using the same equipment employed for δA sampling (a δA sample was always collected concurrent with a δE sample). By maintaining the sampling feed line in the water column and even warmer bottom mud, condensation (and hence isotopic fractionation) was precluded.
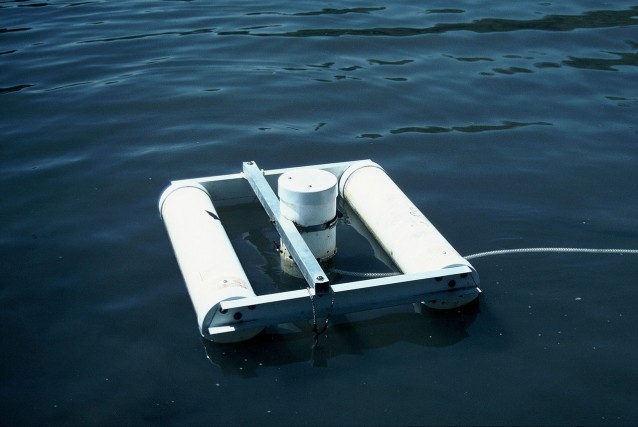
Floating pick up for direct field measurement of the isotopic composition of evaporated lake water δE. Vapour was drawn in from holes about 10mm above the water surface. The sample line was maintained in the warmer water precluding condensation (and isotopic fractionation).
A total of 21 samples were collected over 14 months. Sampling could only be done opportunistically on windless, cold nights, typically between midnight and dawn. Refer thesis for details. Certainly these field experiments were valuable in confirming that directly measured δE is in many cases similar to δE calculated by other means. The experiments provided some confidence that δE derived experimentally from the pan experiments could be applied to the isotopic balance calculations.